Metamaterials: the great possibilities offered by locally resonant media
- matthieurupin
- 11 janv. 2016
- 5 min de lecture
Introduction
Metamaterials have emerged at the beginning of the 2000's following seminal works in both electromagnetic [Ho 1994, Pendry 1996, Pendry 1999] and acoustic fields [Sigalas 1993, Kafesaki 1995, Liu 2000]. Since then, metamaterials have been extended to all kind of

waves. The main idea is based on the consept of sub-wavelength resonators. These objects have the extraordinary capacity of being sensitive to wavelengths greater than their own dimensions (low-frequency resonance).
On the side figure is shown metamaterials for electromagnetic waves (top -- from Schurig 2006) and acoustic waves (bottom --- from Liu 2000). Each time is encapsuled on the top right of the image the unit cell of the structure. For the electromagnetic waves, an open c-shaped wire exhibit a low frequency magnetic resonance. While for the acoustic waves the sub-wavelength resonator is obtained by embedding massic elements (lead sphere) into an elastic matrix (silicon rubber). Note that many other kinds of sub-wavelength resonators exists.
The local resonance induces an interference with an incident wave resulting in a delayed wave at the output. This effect accumulate during the propagation inside the metamaterial and because it occurs at a small scale compare to the wavelength it leads to effective properties for a given wave. Note that the propagation of a wave in a medium is characterized by two parameters: elastic modulus and mass for acoustic waves and electric permittivity and magnetic permeability for electromagnetic waves. Metamaterials offer the possibility to adjust either one of them when using a single type of local resonances or both when mixing resonances [Li 2004].

As a consequence, it is possible to obtain exotic behaviours such as the negative refraction of waves as illustrated on the top image of the figure on the left. It was proposed by J.B. Pendry in 2000 that a slab of negative index material would give an image of an initial source due to negative refraction. But Pendry also pointed out that the evanescent nearfield waves that correspond to high spatial resolution, would be enhanced in the slab. This was recently obtain with acoustic waves on a graphene lattice of Helmholtz resonators (deep sub-wavelength resonators) as shown on the bottom image of the figure on the left (from Kaina 2015).
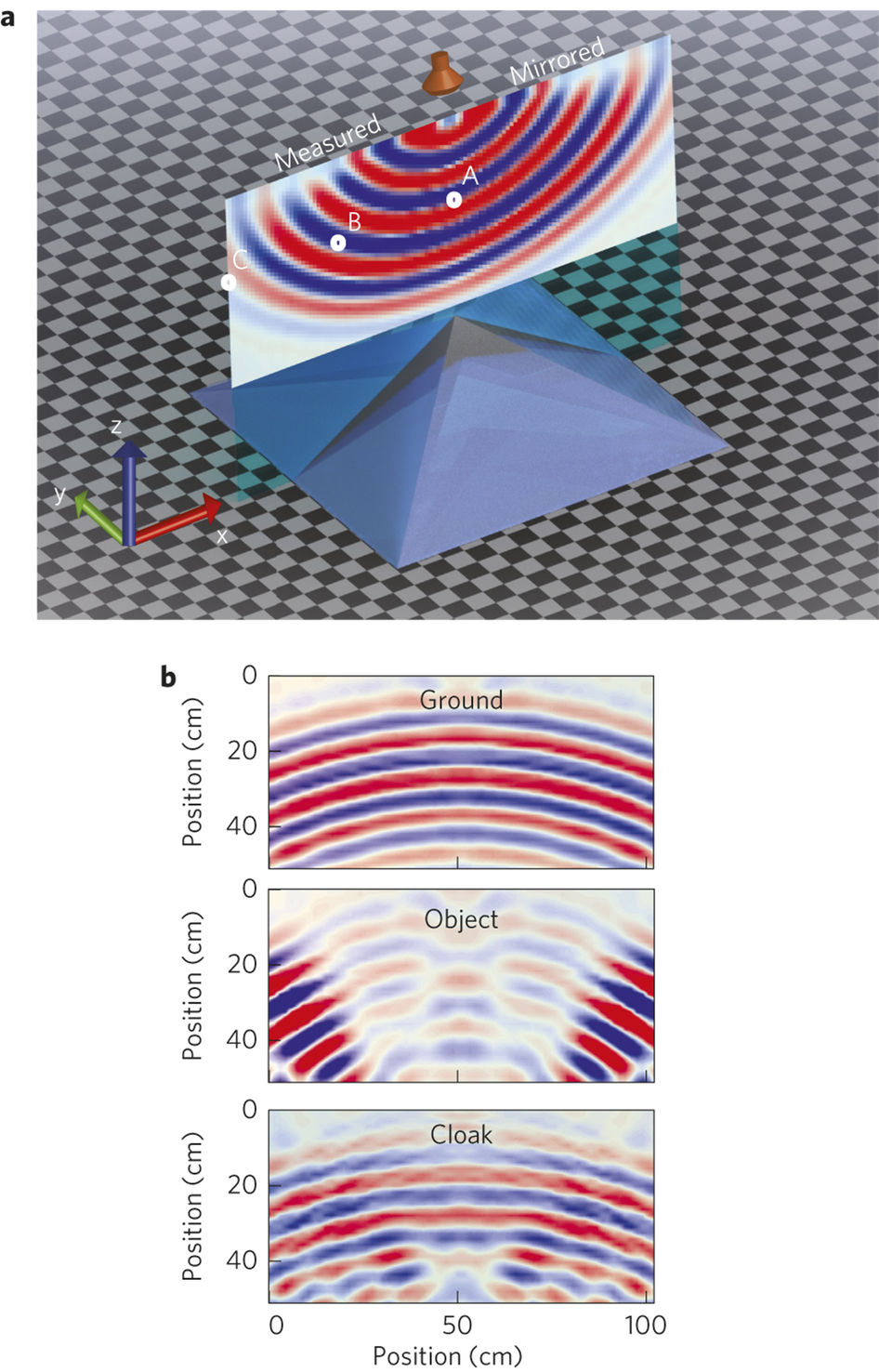
Another extraordinary feature offered by metamaterials is the cloaking of objects. The figure on the right gives an example recently reported of the experimental realization of a 3D invisibility cloak for acoustic waves. A pyramid-shaped assembly of resonant unit cells is placed above an object placed on the ground. The bottom image of this figure gives the spatial (x-z) representation of the instantaneous scattered acoustic field. We see that, with the cloak (bottom), the acoustic wavefield is very similar to the reflection on the ground (top) while the object on its own (middle) presents a strong diffraction signature.
Metamaterials for elastic plate waves
During my thesis I studied metamaterials for elastic plate waves (or Lamb waves) in a Laboratory of Earth sciences (ISTerre, Grenoble, France). The initial motivation was whether we could (should) consider buildings as sub-wavelength resonators for seismic waves. We chose Lamb waves (the name of the symmetric and anti-symmetric elastic waves that propagate in thin plates) for their similarity with seismic waves and because they are suitable for laboratory experiments. So the main object that I studied is the effect on low frequency flexural waves of a set of long metallic rods closely packed at a sub-wavelength scale.
The above galery give an overview of the setup. A table-size aluminum plate of 6-mm-thick is decoupled from a support in order to minimize the energy leakage. The flexural waves generated by a vertically arranged shaker are trapped in the plate which produces very long reverberated signals (codas). The measurement is realized with laser doppler vibrometers and I studied two different arrangement of rods: random and periodic. We demonstrated that the strong perturbation caused by the rods over the propagation of Lamb waves is not due to their periodic arrangement. Similarly, such a metamaterial present an isotropic behavior explain by the symmetry of the resonances. We also use this configuration to perform super-focusing experiments. And finally we pointed out the particularly complex hybrization effect created by the flexural resonances of the rods which break the natural orthogonality of the symmetric and anti-symmetric zero-order Lamb modes.
Currently, Philippe Roux (ISTerre) and I are trying to study experimentally the particularly interesting effects of symmetric metamaterials on Lamb waves.
Active metamaterials with a gradient of index to mimic cochlear wave
During my post-doctorat at the Institut Langevin I am studying the possibility offered by the metamaterials to reproduce the pecular behaviour of the cochlear wave. This study involves metamaterials which present a continuous gradient on their unit cells resonances adding with active feedback loop necessary to mimic the non-linear response of the cochlea. This is work is in progress and I am currently writing articles on this subject. For this reason I will just describe the particularity of the system and I will complete this post after the publication of my results.
The left figure of the above gallery illustrates briefly the physiological aspects of the cochlea. a) Overview of the inner ear (vestibular system + cochlea). b) The cochlea is crossed by 3 channels. The cochlear duct, filled with endolymph (pink), is surrounded by the scala vestibuli (above) and the scala tympani (below) which are themselves filled with perilymph (blue). These fluids have different ionic compositions for the actuation of molecular motors which boost the capabilities of our ear (active system) especially at low level of incoming sound. c) The walls of the cochlear duct are two elastic membranes: Reissner's membrane (above) and the basilar membrane (below). d) When a sound is detected, it starts to vibrate the basilar membrane to which is attached the organ of Corti. The latter is responsible for the mechanico-electrical transduction through the hair cells. Further information may be obtained from the http://www.cochlea.eu website.
The right figure of the above panel describes the property of the wave travelling in a dead cochlea (passive). The basilar membrane is a one-dimensional system that is vibrated by the action of the staps. The latter is a small bone of the middle ear which is in contact with an elastic membrane of the cochlea called the oval window (not visible here). The fluid in the scala vestibuli is then vibrated. However, as schematically illustrated in Figure (b), it should be noted that the fluids of the scala tympani and the scala vestibuli communicate through an orifice at the apex of the basilar membrane. Thus, the fluid located below the membrane (in the scala tympani) has a movement opposed to that of the one of the scala vestibuli. It causes the vibration of the basilar membrane. The response of the latter is then very remarkable. Indeed, a monochromatic vibration has a maximum located at a particular point on the membrane which depends on the frequency of the excitation. This behavior is explained by the continuously evolving nature of its mechanical parameters (stiffness and density). The membrane is narrow and thick at the base, which corresponds to local resonances of high frequencies. While at the apex is enlarged and fine to code the low frequencies.
This configuration is reproducible with a metamaterial having continuously evolving local resonances. I will demonstrate this in more detail soon after the publication of my articles in preparation.
Comentarios